Accurate ligand affinity determination: high-affinity ligands for use in cancer immunotherapy
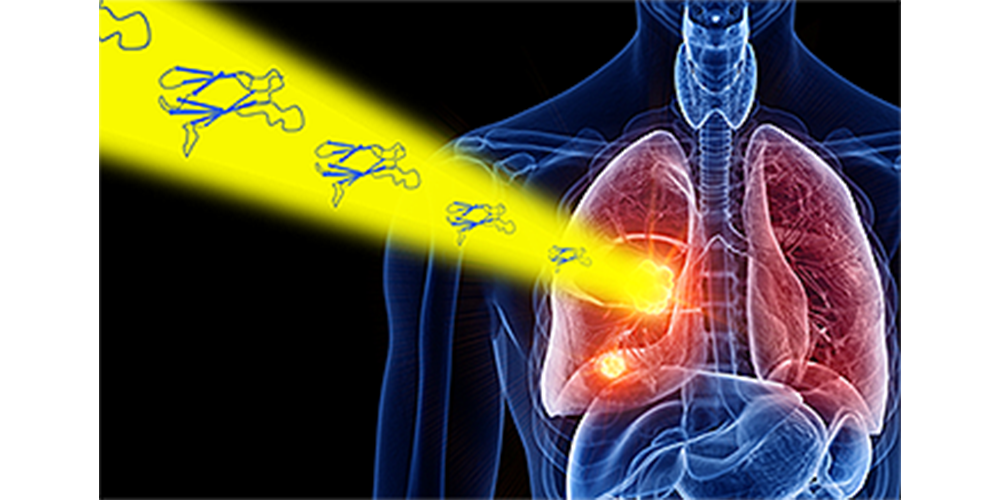
Johan Engström, Sr Scientist at Gyros Protein Technologies
Part 1: High-affinity ligands for imaging target expression in cancer immunotherapy
Cancer immunotherapy targeting protein-protein interactions saves lives. Success builds on the binding characteristics of high-affinity binding therapeutic antibodies and also ligands used to monitor target expression during clinical trials. Measuring nano- to low-picomolar affinity binding can be difficult using surface plasmon resonance (SPR), which led a biotherapeutics discovery team to choose in-solution affinity analysis in the characterization a high-affinity imaging ligand.
I want to tell you about a recent paper from Bristol Mayer Squibb that links together Nobel prize-winning developments in cancer immunotherapy and the need to measure affinity of designer ligands at a level that tests the limits of many analytical methods. It all starts with targeting protein-protein interactions.
Early efforts in drug design focused almost entirely on small molecules to target well-defined protein–ligand interactions, but limitations in this approach sparked an interest in targeting malfunctioning protein-protein interactions (PPI) that can lead to many diseases. This opened up a whole new world of possibilities with high-affinity ligands including antibodies, antibody derivatives, and peptides to target some of the estimated 130,000–650,000 types of PPIs in the human interactome (1). Some of these PPIs involve cancer progression.
Cancer immunotherapy – an example of how targeting PPIs saves lives
Immunotherapy has been described as the new fourth pillar of cancer treatment, after surgery therapy, chemotherapy, and radiation therapy. While cancer immunotherapy goes back to the efforts of William Coley in the late nineteenth century (2), it is only recently that the real power of this approach has become apparent, with pioneering work by, for example, James P. Allison and Tasuku Honjo that led to their 2018 Nobel Prize in Physiology or Medicine. These researchers revealed the importance of immune checkpoints that act as ‘brakes’ on the immune system, and the inhibition of these ´brakes´ enables T cells to more effectively eradicate cancer cells (3). And this isn’t just a question of increasing median survival. It can actually cure cancer, as Allison pointed out when he talked about his emotional meetings with cancer patients who had been cured thanks to his efforts.
Immune checkpoint inhibitors in immunotherapy
The example of immunotherapy that we will follow involves suppressing the binding of the programmed death-ligand 1 (PD-L1) on tumor cells to programmed cell death protein 1 (PD-1) on the surface of T cells that inactivates the T cells, enabling the cancer cells to escape detection by the immune system and attack by the T cells. Leading this effort, Bristol Myers Squibb (BMS) launched the first PD-1 immune checkpoint inhibitor to block the PD-1/PD-L1 interaction, the antibody Opdivo (nivolumab), which received regulatory approval in 2014. There are currently five approved antibodies on the market (4).
The need to image PD-L1 using smaller molecules
While immunotherapy targeting the PD-1/PD-L1 pathway has delivered impressive clinical results, not all patients respond sufficiently and may even show immune-related adverse events following treatment. The response depends on the PD-L1 expression levels in the tumor, and this can be imaged using real-time, non-invasive positron emission tomography (PET) together with radiolabeled antibodies targeting PD-L1. The images are then used to monitor the therapeutic response and optimize drug therapy. Antibodies as PET ligands have several disadvantages however, including long circulating half-lives that limit clinical workflow, the large molecular weight that can impede targeting, and Fc domains that can activate antibody-dependent cellular cytotoxicity (5). These limitations motivated the team at BMS to search for smaller molecules with improved pharmacokinetic profiles (6, 7).
Adnectins: low complexity antibody-mimics with comparable affinity and specificity
The success of therapeutic monoclonal antibodies stimulated a search for simpler molecules that retain the tight and specific target binding, low toxicity and low immunogenicity of antibodies, but are easier to discover and more readily manufactured at lower cost. One development was Adnectins, a family of proteins based on the 10th fibronectin type III domain that binds with high affinity and specificity to therapeutically relevant targets (6). While they share motifs with the variable domains of antibodies and have comparable binding affinity and specificity, Adnectins have a simpler structure that is easier to manipulate
Overcoming the challenge of analyzing high-affinity ligands
BMS used Adnectin-based technology to develop PET ligands directed against PD-L1, resulting in BMS-986192 (5), which was used to detect PD-L1 in mouse tumors and in non-human primate studies. This ligand is now used in ongoing clinical trials in patients undergoing immunotherapy. One challenge the Discovery Biotherapeutics team at BMS faced was measuring the high affinity of Adnectins prepared in different formats. In earlier work using surface plasmon resonance (SPR) kinetic measurements, the equilibrium dissociation constant (KD) could only be estimated to <35 pM in since the dissociation rate is close to the detection limit of the instrument. As we will see in the next post in this series, achieving a more accurate determination of the affinity required switching to a new methodology based on in-solution affinity analysis using the Gyrolab® technology (9).
References:
- Recent advances in the development of protein–protein interactions modulators: mechanisms and clinical trials. Lu H. et al. Signal Transduction and Targeted Therapy 2020 5:213.
- Coley’s toxins in perspective. Starnes CO. Nature (1992) 357:11–2. doi:10.1038/357011a0.
- Nature Collection October 2018: Nobel Prize in Physiology or Medicine 2018.
- PET Imaging of Tumor PD-L1 Expression with a Highly Specific Nonblocking Single-Domain Antibody Gaochao Lv et al. J Nucl Med 2020; 61:117–122 DOI: 10.2967/jnumed.119.226712.
- Molecular imaging for cancer immunotherapy. Lim E. A. et al. 2020. Immuno-Oncology Technology 5, 10–21.
- Immune Checkpoint PD-1/PD-L1: Is There Life Beyond Antibodies? Konstantinidou M et al. Angew Chem Int Ed Engl. 2018 April 23; 57(18): 4840–4848. doi:10.1002/anie.201710407.
- Synthesis and biologic evaluation of a novel (18)F-labeled adnectin as a PET radioligand for imaging PD-L1 expression. Donnelly DJ et al. J Nucl Med. 2018 59:529–535.
- Adnectins: engineered target-binding protein therapeutics. Lipovšek, D. Protein Engineering, 2011 Design & Selection vol. 24 no. 1–2 pp. 3–9.
- Application of the Gyrolab microfluidic platform to measure picomolar affinity of a PD-L1-binding Adnectin™ radioligand for positron emission tomography. Dai Z. et al. BioTechniques 2020. 69: 00–00 10.2144/btn-2020-0080.